Unless you’ve been living under a rock for the past decade, the emergence of wearable tech should not be an alien concept. Wearable smart devices have evolved and now monitor everything—from your activity during the day to those precious z’s at night. Sleep is essential to our physical and mental well-being; our bodies use this time to repair and rejuvenate cells and clear toxins away.
Let’s look beneath the surface and explore the essential function of sleep and the activities that take place in our bodies during slumber. Then, let’s delve into wearable tech in healthcare the science behind the latest devices and the advancements in sleep tracking.
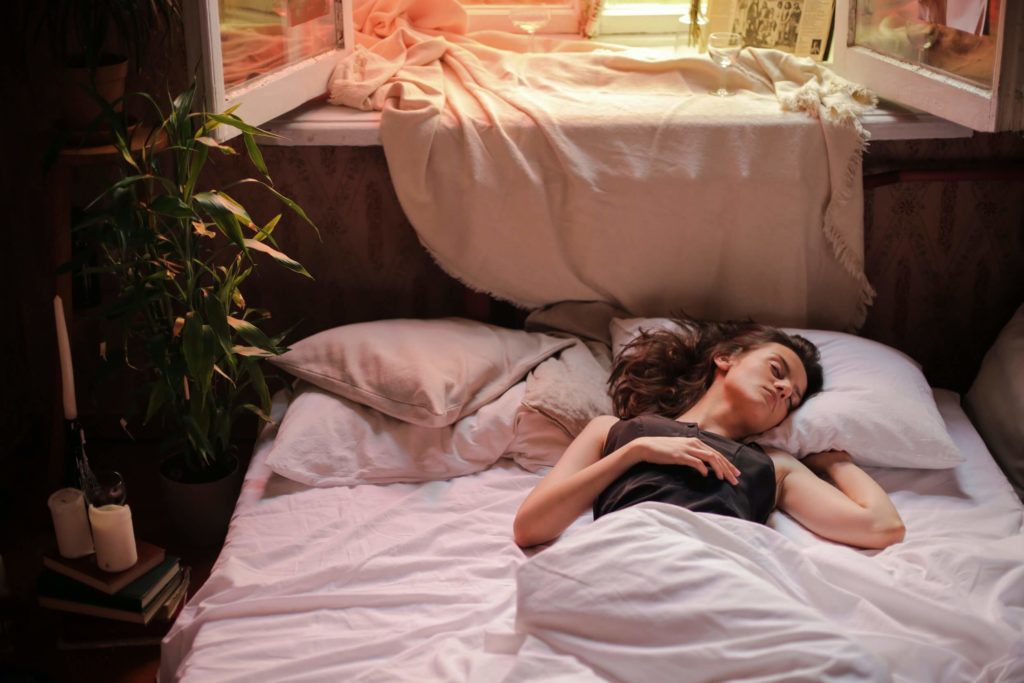
Highlights
- Our sleep-wake cycle is controlled by our circadian rhythm, an internal biological clock that interprets the environment and controls hormone release to dictate our behaviour,
- Wearable technology uses sensors to track important parameters in individuals while they sleep—such as heart rate and blood oxygen(O2) levels,
- There are two potential drawbacks of wearables—lack of standardisation of metrics and accuracy compared to the gold-standard PSG sleep test.
The Science of Sleep: What Happens to Your Body When You Sleep
Sleep is a vital biological function known to be correlated to human health and performance, but the inner workings of the body, while we sleep, have not been fully understood yet. We sleep for a third of our day (ideally), during which several biological processes occur in our bodies: cellular restoration—whereby our cells and muscles repair themselves—protein synthesis and tissue growth.
Our glymphatic system—responsible for the distribution of compounds and eliminating waste throughout the brain—comes alive when we sleep. It eliminates toxic byproducts such as soluble proteins and metabolites (end product of metabolism) that accumulate in the brain, gearing us up for the next day. Sleep is known to play an integral role in our metabolism and a lack of sleep wreaks havoc on our systems.
Two Biological Mechanisms Control our Sleep-Wake Cycle
- The Circadian rhythm
- Homeostasis
1. Circadian rhythms
Our 24-hour internal biological clock, control metabolism and hormone release. Environmental changes, such as temperature and light, trigger appropriate responses—they keep us alert during the day and sleepy at night. Our tissues and systems (endocrine, digestive, etc.) have secondary internal clocks—or ‘peripheral oscillators’—which send messages to the brain that help trigger our sleep-wake cycle.
Circadian rhythms modulate the release of numerous hormones, including:
- Melatonin is the hormone that induces sleep.
- Cortisol, the stress hormone, decreases while we sleep at night and peaks just after we wake up.
- The growth hormone, released by the pituitary gland, helps cells in our bodies repair and grow while we’re asleep.
- Antidiuretic hormone, which switches off the need to urinate during the night.
- Proteins called cytokines are released by our immune system, which helps prevent inflammation.
The next time you binge-watch Netflix late into the night, snacking on your favourite munchies, know that you might be throwing your circadian rhythm out of whack.
Blue light from screens suppresses melatonin, causing an increase in heart rate. You can use dark-tinted blue light-blocking glasses to help, but it’s a good idea to avoid screens before bedtime and create a relaxing pre-bedtime routine.
Moreover, you need to digest your food properly before the stomach calls it a day.
As a result of doing all this extra work, your body takes longer to cool down to an appropriate temperature to get some sleep (a couple of degrees below normal).
2. Homeostasis
A state of internal equilibrium, tracks our need for sleep, regulates its intensity and reminds our bodies to get some solid sack time. Our ‘sleep drive’ or desire to sleep, increases the longer we are awake and is linked to the release of adenosine.
Adenosine is a neurotransmitter that inhibits brain processes associated with being alert and awake. It is also a natural painkiller and regulates our heart rhythm. Adenosine levels in our bodies gradually increase throughout the day and peak at bedtime, prompting sleepiness.
What are the 4 cycles of Sleep?
When we nod off, a series of stages—four, to be precise—puts us into a deep sleep, characterized by changes throughout the body. They are:
- Non-rapid Eye Movement
- Light Sleep
- Deep Sleep
- REM – Rapid Eye Movement
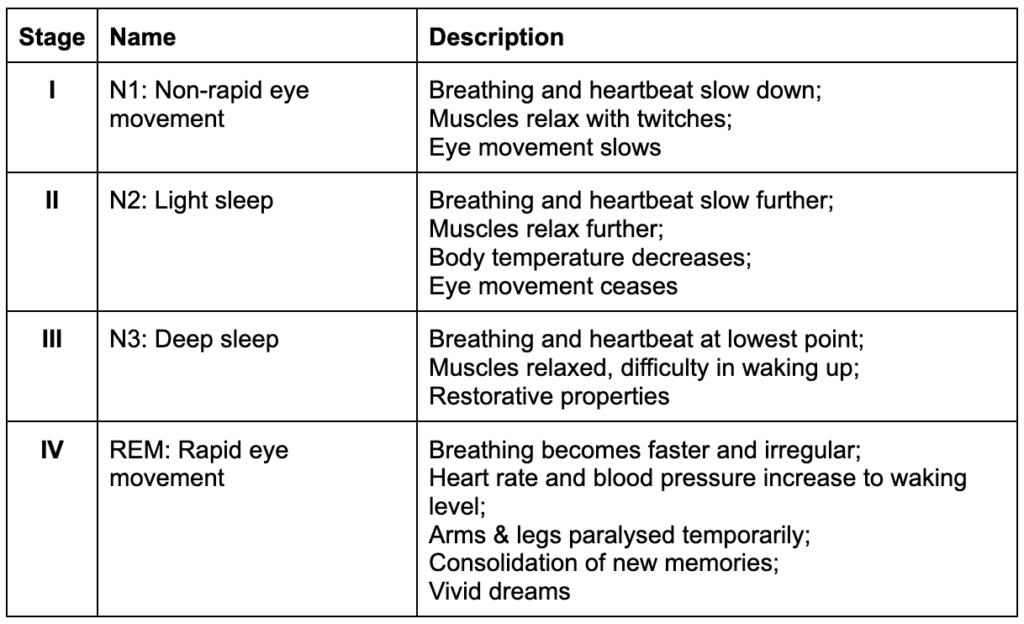
Each cycle (from stage I to IV) lasts around 90 minutes. If awoken after a completed cycle, one is more refreshed than if awoken during it.
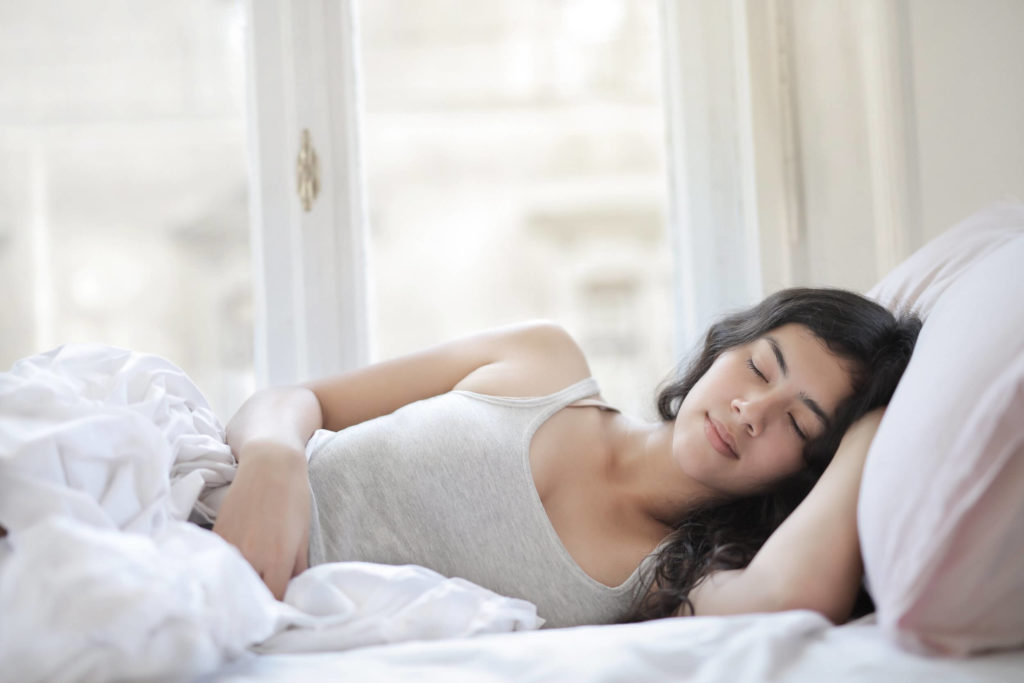
Neurological Brain Activity While Asleep
What transpires in our brains while we sleep? Various regions are surprisingly active during the four stages:
- Within the hypothalamus lie clusters of cells called the suprachiasmatic nucleus (SCN), the control centre of our circadian rhythm.
- In low light, the pineal gland produces melatonin and distributes it through the bloodstream and cerebrospinal fluid.
- The basal forebrain releases adenosine, which increases sleep drive.
- The brain stem (pons, medulla and midbrain) controls transitions between wake and sleep. It communicates with the hypothalamus and other regions of the brain.
- The thalamus is a region that relays sensory information to our brain. It is inactive during the first three stages but becomes active during REM sleep.
- The thalamus communicates with the cerebral cortex, the covering of the brain responsible for processing and interpreting information, learning and memory. The thalamus sends images, sounds and other sensations to the cerebral cortex when we are dreaming.
- When we fall into REM sleep, the amygdala becomes active, helping us process emotions while we dream. Dreaming occurs for approximately two hours each night and is most vivid during REM sleep.
An effective way of tracking brain activity during sleep is by measuring the frequency of brainwaves (in Hertz or Hz). Brainwaves are measured using an electroencephalogram (EEG), which helped researchers categorise sleep into the four distinct stages we understand today. When we are awake and alert, our brainwaves operate at gamma, a frequency between 12 and 35 Hz.
In stage N1, brainwaves are smaller and more uniform (than gamma) and slow to a frequency between alpha (8-12 Hz) and theta (4-8 Hz).
During N2, brain waves continue to slow, but are prone to occasional bursts of rapid waves, dubbed sleep spindles. As our bodies enter the deep, restorative state of N3, brain waves slow to delta, a frequency between 0.5 and 4 Hz. If we don’t get enough sleep with delta waves, it leads to an inability to rejuvenate the body and revitalise the brain.
When we enter REM sleep, our brainwaves suddenly spike in frequency (to gamma levels) as if we are awake. This suggests heightened activity in our central nervous system and is thought to play an important role in information processing and memory creation.
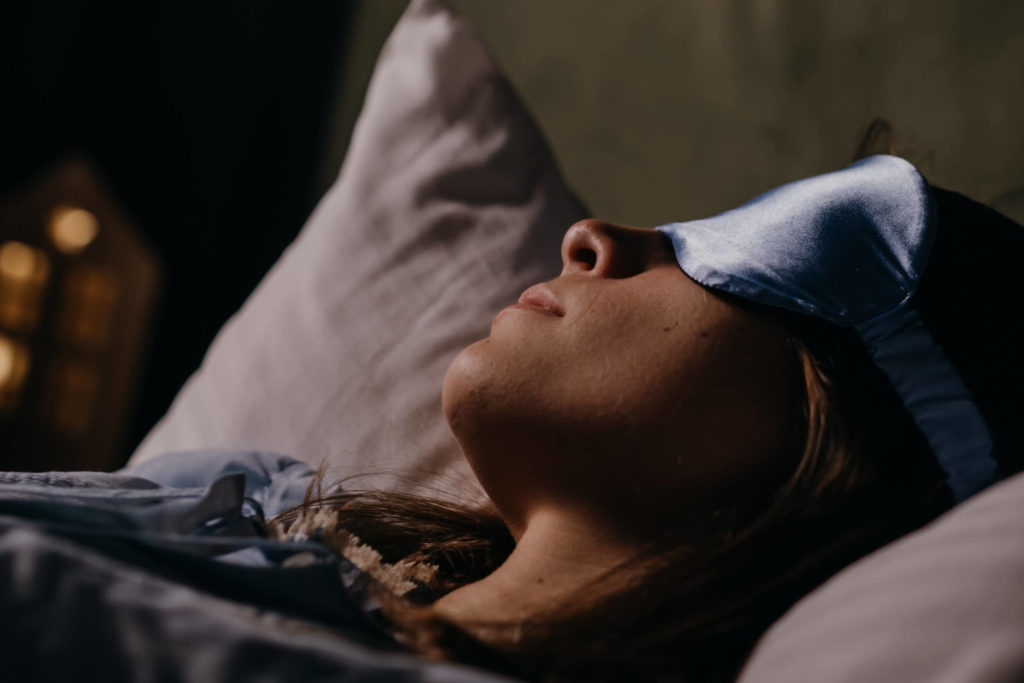
The Science Behind Fitness Wearable Tech
Referred to as the gold standard for studying sleep, polysomnography (PSG) continues to be the benchmark for sleep evaluation. A comprehensive test that monitors an individual’s brain waves (EEG), blood O2 level, resting heart rate, muscle activity and breathing, it is used to diagnose sleep disorders. However, PSG is an expensive and cumbersome clinical study, not feasible for the masses.
The advent of several sleep-sensing technologies has changed the landscape of studying sleep, the ultimate goal being continuous sleep monitoring. Manufacturers are making sensors more accurate, smaller, and less intrusive for the user.
Technologies include bed sensors, mobile health, radio frequency (RF) sensors, ultrasound, wireless EEG, and smartwatches or wearable tech. Sleep measurement devices can now monitor peoples’ brain waves, oxygen level, circadian rhythms, heart rate, respiratory rate, and body temperature.
A study by Pozuelo et al charted the accuracy versus usability (user burden) of sleep measurement devices, and it emerged that wearable devices and bed sensors offer the least user burden with moderately high accuracy (PSG has higher accuracy but with a much higher burden on the user).
Researchers compared devices across a range of metrics, including their measurement of sleep time, quality, and stage. They also evaluated the technology based on its scalability and usability (user burden).
Wearable tech emerged as a fairly accurate solution to collect data en masse. In an age where data is king, researchers can effortlessly collect user data across long time periods for a wide demographic (called free-living studies).
Data begins its life in a clinical study or from your wearable device. Once it is stored and uploaded to a server, processed, cleaned, and modelled, it has an abundance of applications.
Apart from use in longitudinal (observational, long time-period) studies and research, this data has numerous uses in the health and wellness industry. Doctors and nurses can make recommendations based on a patient’s past data and consumers may benefit from lifestyle recommendations after their devices notice trends in their behaviour.
Insurance and pharmaceutical companies can view client data to monitor how their clients are doing and whether any adjustments need to be made.
As with any emerging technology, challenges remain. For one, there is no standardisation across products—no defined or accepted guidelines for this plethora of products to adhere to.
There is an inherent lack of technical information disclosed by companies, such as sensor accuracy and algorithms used, which is somewhat understandable, given that these are trade secrets or intellectual property of the companies themselves.
However, it does not provide an apples-to-apples comparison and therein lies the problem.
Secondly, the reliability of data must come into question. Is the metric being captured accurately? Will the devices exhaustively capture all important data?
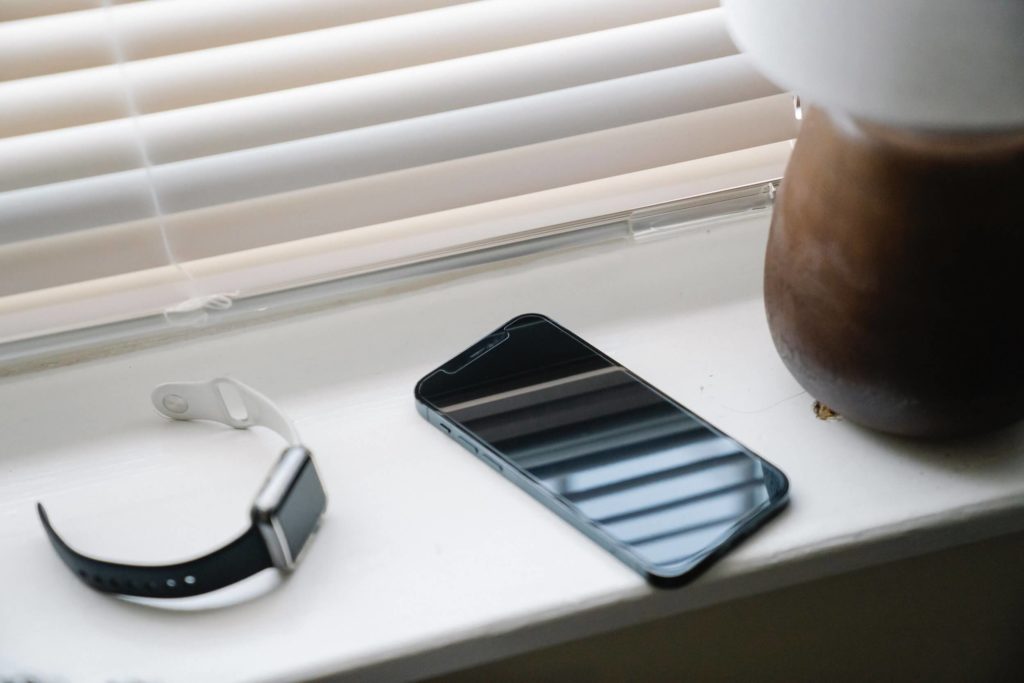
How does wearable tech track your sleep?
So how do these wearable devices accurately track our sleeping habits? What technology is at play?
Wearable technology is any electronic device worn on a user’s body and includes medical devices, accessories, jewellery, and clothing. Devices are equipped with a combination of sensors, each with its own function.
Actigraphy
Actigraphy, most commonly used in healthcare wearable technology, is a validated method of study that uses monitors on the wrist or ankle to record the acceleration or deceleration of body movements or sleep disorders.
Actigraphy relies on accelerometers which, as the name suggests, are sensors that measure the acceleration of a body or structure.
Accelerometers
Accelerometers use movement data to make predictions of the time spent asleep versus awake and the current sleep stage. A device that measures the vibration, or acceleration of motion of a structure.
Sensors track basic human movements such as body orientation, tilt, incline and rate of speed change (of the body) throughout the day.
Actigraphy and accelerometry both have potential drawbacks, including a lack of standardisation of human activity recognition (for example, device A may have a different reading than device B for the same activity) and a lack of assessment techniques for sleep during the day.
Smartwatches and wearable devices use a combination of actigraphy and heart rate monitors to derive their metrics. These metrics are displayed on an accompanying smartphone app, where users can view their historical data.
Henriksen et al. conducted a study of 423 fitness trackers and smartwatches released from 2011 to 2017, each equipped with different sensors, algorithms and mobile apps for viewing and analysing data. They noticed that sensor support improved every year and that the most common sensors found were the accelerometer and the photoplethysmogram (used to estimate heart rate). Photoplethysmography uses light to track blood flow volume changes.
To track sleep, a host of other sensors have made their way into wearable devices, such as BP monitors, mini ECGs and pulse oximeters. ECG sensors use electrodes to detect small electrical impulses emitted with each heartbeat.
Due to COVID-19, we are no strangers to pulse-oximeters—they measure our blood oxygen levels with fair accuracy. Mobile phones use a combination of gyroscopes, accelerometers and microphones to chart our movement while asleep.
Gyroscopes
Gyroscopes follow the principle of conservation of angular momentum to measure angular velocity and are used in compasses, aircraft and ships to detect the deviation of an object from its intended orientation.
Gyroscopes detect our body orientation and rotation while we sleep.
The caveat with using mobile phones to track sleep, however, is that the device must be in close proximity to the user to be able to measure sleep metrics.
It is important to note that while assessing data, one must be careful of ‘noise’ or extraneous data. Aggregating sleep data from various sources (i.e., more than one device) may solve this by making models more robust and tolerant to ‘noise’ and/or missing data.
Rings, which are one of many types of sleep trackers, can track pulse rate, a variation on inter-beat intervals (IBIs) and pulse amplitude. A study conducted by Zambotti et al compared one of the ring models to traditional PSG. Although the model detected some stages of sleep with unerring accuracy, in other states the results were mixed, indicating that the field has some way to go to replicate results from a PSG.
Reader, beware. In some cases, adopters of wearable sleep monitors suffer from orthosomnia, a condition where users suffer poor quality sleep because they are worried about achieving a high ‘sleep score.’ In these instances, individuals must improve their sleep hygiene to achieve better sleep.
Conclusion
Sleep is as vital to our bodies as sustenance; a lack of sleep increases our risk of cardiovascular disease, obesity, high blood pressure and diabetes. Our circadian rhythm is a 24-hour biological clock that dictates when we go to bed. However, all the biological processes that underpin sleep have not been fully explored. To date, the most accurate method of studying sleep is polysomnography (PSG), which has enabled us to identify its four distinct stages. Our systems behave distinctly during sleep, identified by parameters such as heart rate, respiratory rate and movement.
Enter wearable devices, an unobtrusive method of measuring sleep across a wide range of demographics and over a long period of time. As an emerging technology, there is a gulf in accuracy when comparing wearables to PSG testing (which is more comprehensive and expensive). Companies use different sensors such as accelerometers and photoplethysmograms to measure everything from movement to heart rate. They then create their own algorithms to determine sleep metrics for individuals, leading to a lack of standardisation across devices in the industry. If companies share raw data, it serves to fuel further research in the field and has a host of end-user applications in the health and wellness space.
Disclaimer: The contents of this article are for general information and educational purposes only. It neither provides any medical advice nor intends to substitute professional medical opinion on the treatment, diagnosis, prevention or alleviation of any disease, disorder or disability. Always consult with your doctor or qualified healthcare professional about your health condition and/or concerns and before undertaking a new health care regimen including making any dietary or lifestyle changes.
References